What are 5 engineering methods?
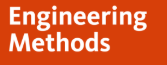
In the ever-evolving landscape of technological advancement and global challenges, the role of engineers has become increasingly crucial. As the world grapples with complex issues ranging from sustainable energy and climate change to healthcare and urban infrastructure, engineers have emerged as the driving force behind many of the most innovative and impactful solutions.
At the heart of this engineering prowess lies a diverse array of problem-solving methodologies and techniques – collectively known as engineering methods. These systematic, structured approaches to problem-solving have been refined and honed over centuries, drawing upon the principles of science, mathematics, design, and systems thinking.
In this comprehensive article, we will explore the various engineering methods that have transformed the way we tackle complex challenges and drive progress. From the foundational scientific method to the emerging paradigms of computational engineering and green engineering, we will delve into the key characteristics, applications, and transformative potential of these powerful problem-solving frameworks.
By understanding the versatility and adaptability of engineering methods, we can gain valuable insights into how engineers navigate the complexities of the modern world and contribute to a more sustainable, equitable, and technologically advanced future.
The Scientific Method: The Cornerstone of Engineering
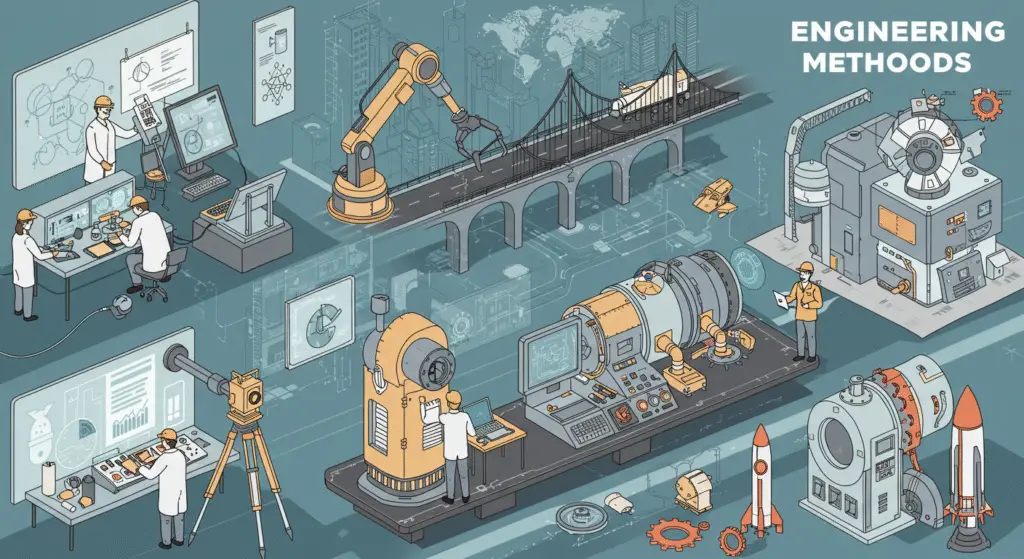
At the core of the engineering discipline lies the scientific method – a systematic and empirical process for investigating phenomena, testing hypotheses, and drawing conclusions based on evidence. This problem-solving approach, which dates back to the 17th century and the groundbreaking work of scientists like Sir Isaac Newton and Francis Bacon, has been a foundational pillar of engineering practice.
The five key stages of the scientific method are:
- Observation and Problem Identification: The process begins with observing the world around us, identifying patterns, and recognizing the existence of a problem or question that requires further investigation.
- Hypothesis Formation: Based on the initial observations, researchers formulate a testable hypothesis – a proposed explanation for the phenomenon or problem at hand.
- Experiment Design and Data Collection: The next step involves designing and conducting experiments or studies to collect relevant data that can be used to test the hypothesis.
- Data Analysis and Interpretation: The collected data is then analyzed, often using statistical methods, to determine whether the hypothesis is supported or refuted by the evidence
- Communication and Revision: Finally, the findings are communicated to the scientific community, and the hypothesis may be revised or refined based on feedback and new insights.
The scientific method’s emphasis on empirical observation, hypothesis testing, and the iterative refinement of knowledge has made it a cornerstone of scientific discovery and technological innovation. By following this structured approach, engineers can develop a deeper understanding of the problem, generate evidence-based solutions, and continuously improve their understanding and approach.
The scientific method is widely applied in various engineering disciplines, from mechanical and electrical engineering to civil and environmental engineering. Whether tackling a complex design challenge, developing a new product, or optimizing an existing system, engineers rely on the core principles of the scientific method to ensure their solutions are grounded in empirical evidence and rigorously tested.
Computational Engineering: Harnessing the Power of Digital Tools
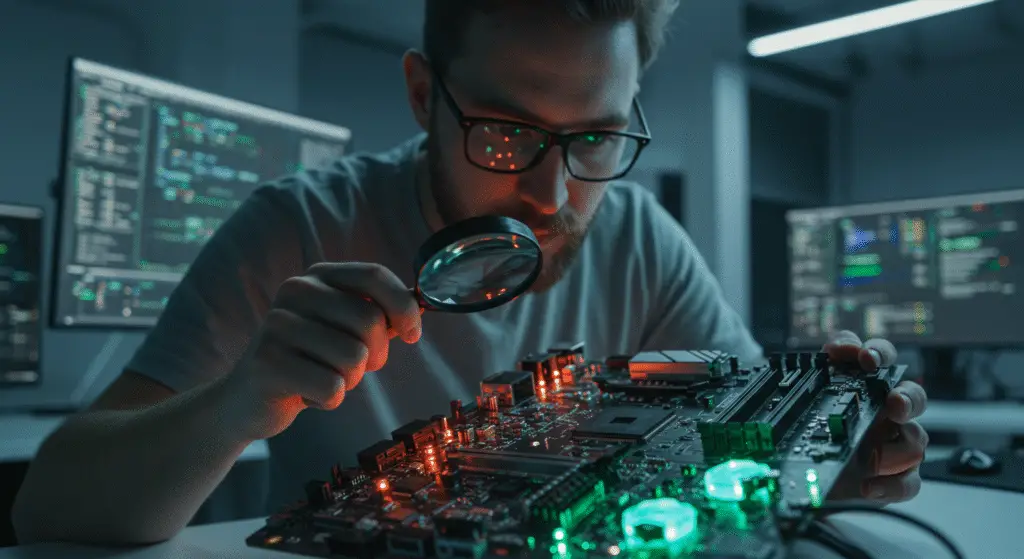
As the world has become increasingly digitized, the field of engineering has undergone a transformative shift, with the rise of computational engineering – the integration of powerful computational tools, simulations, and data analytics into the problem-solving process.
The advent of high-performance computers, sophisticated software, and advanced algorithms has fundamentally changed the way engineers approach their work, enabling them to tackle increasingly complex problems with greater speed, accuracy, and efficiency.
Key elements of computational engineering include:
- Computational Modeling and Simulation: Engineers leverage computational fluid dynamics, finite element analysis, and multiphysics simulations to model and analyze complex systems and processes with unprecedented precision.
- Data-Driven Decision-Making: The integration of big data, machine learning, and artificial intelligence empowers engineers to uncover hidden patterns, predict system behaviors, and make data-driven decisions that improve the performance, reliability, and resilience of their designs.
- Virtual Prototyping and Optimization: Computational tools enable engineers to explore a wider range of design alternatives, simulate various scenarios, and optimize solutions in the virtual realm, reducing the need for physical prototyping and accelerating the innovation process.
- Rapid Iteration and Feedback Loops: The digital nature of computational engineering allows for rapid iterations, quick testing, and the seamless incorporation of feedback, enabling engineers to refine and improve their solutions in real-time.
By embracing computational engineering, engineers can enhance the accuracy of their analyses, reduce development costs and timelines, and tackle engineering challenges that were previously intractable. This digital transformation has not only improved the efficiency and effectiveness of engineering practices but has also paved the way for the development of increasingly sophisticated and innovative products and systems.
As computational power continues to grow, and the integration of emerging technologies like artificial intelligence and virtual reality becomes more prevalent, the field of computational engineering is poised to play an increasingly pivotal role in shaping the future of engineering.
Green Engineering: Designing for Sustainability and Resilience
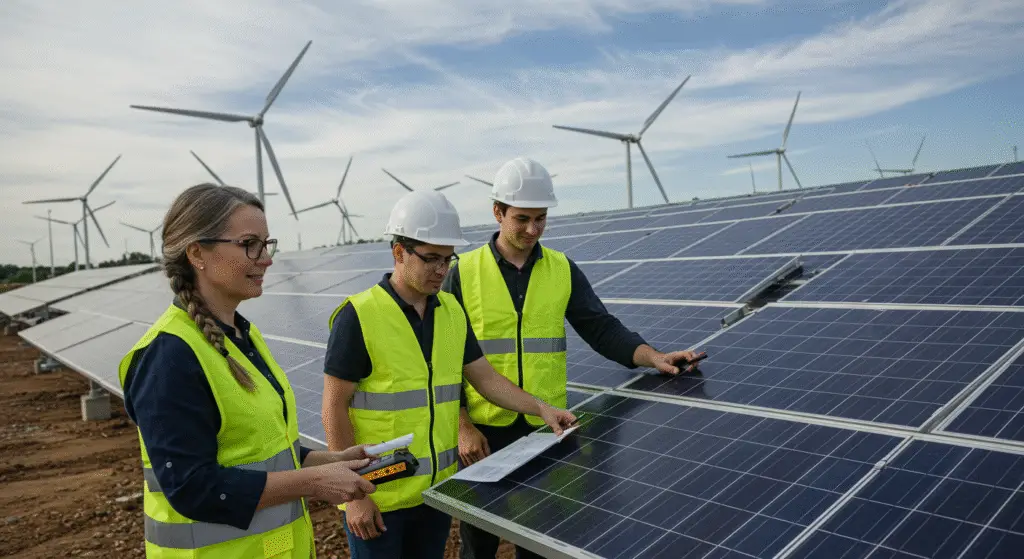
Alongside the advancements in computational engineering, the field of green engineering has emerged as a critical contributor to the growing emphasis on sustainability and environmental stewardship in the engineering discipline.
Green engineering, or sustainable engineering, is a problem-solving approach that focuses on designing products, processes, and systems that minimize environmental impact, conserve natural resources, and promote the well-being of both human and natural ecosystems.
Key elements of green engineering include:
- Life-Cycle Assessment: Green engineers consider the environmental impact of a product or system throughout its entire life cycle, from raw material extraction to disposal or recycling.
- Renewable Energy and Energy Efficiency: The development of renewable energy technologies, such as solar, wind, and energy storage systems, as well as the integration of energy-efficient designs, are central to green engineering.
- Circular Economy Principles: Green engineers embrace the principles of the circular economy, aiming to design for reuse, repair, and recycling, minimizing waste and promoting the regeneration of natural systems.
- Biomimicry and Nature-Inspired Design: By drawing inspiration from natural systems and processes, green engineers develop innovative solutions that are aligned with ecological principles and resilient to environmental changes.
- Interdisciplinary Collaboration: Green engineering often involves close collaboration with experts from various fields, including environmental science, policy, and community engagement, to ensure that engineered solutions are responsive to the diverse needs and concerns of stakeholders.
The emergence of green engineering has been a direct response to the pressing global challenges of climate change, resource depletion, and environmental degradation. By leveraging the latest technologies and embracing a holistic, systems-level approach, green engineers are poised to play a pivotal role in addressing the environmental challenges of our time and shaping a more resilient and sustainable future.
The Engineering Method: A Systematic Approach to Problem-Solving
At the core of the engineering discipline lies the engineering method – a structured, logical, and iterative process that engineers employ to identify, analyze, and solve complex problems. This systematic approach, which draws upon the principles of science, mathematics, design thinking, and systems analysis, has been instrumental in driving technological innovation, improving human welfare, and shaping the world around us.
The key stages of the engineering method are:
- Problem Identification and Framing: Clearly defining the problem or opportunity at hand, gathering relevant information, and understanding the context and constraints.
- Research and Analysis: Conducting in-depth research to understand the problem, analyzing existing solutions and best practices, and developing mathematical models and simulations.
- Ideation and Concept Generation: Generating a diverse range of potential solutions, evaluating their feasibility and effectiveness, and collaborating with interdisciplinary teams.
- Design and Optimization: Selecting the most promising concepts, designing the technical details and specifications, and continually refining and optimizing the solution through testing and iteration.
- Implementation and Testing: Constructing and deploying the engineered solution, collecting data, and conducting rigorous testing to validate its performance and effectiveness.
- Evaluation and Iteration: Assessing the solution’s impact, gathering feedback from stakeholders, and continuously improving the solution based on new insights and lessons learned.
The engineering method’s emphasis on scientific thinking, mathematical reasoning, and systems-level analysis makes it particularly well-suited for tackling complex, technological challenges. However, its versatility also allows it to be applied to a wide range of problems, from social and environmental issues to organizational and business challenges.
By integrating ethical considerations, fostering multidisciplinary collaboration, and embracing the convergence of emerging technologies, the engineering method is poised to play a pivotal role in shaping a more sustainable, equitable, and technologically advanced future.
The Systems Thinking Approach: Navigating Complexity
In an increasingly interconnected and interdependent world, many of the challenges we face are characterized by complexity, dynamism, and the interplay of multiple, interrelated components. In this context, the systems thinking approach has emerged as a powerful problem-solving framework that enables engineers to navigate these intricate challenges.
The core principles of the systems thinking approach include:
- Holistic Perspective: Systems thinkers view problems as part of a larger, interconnected system, rather than as isolated, standalone issues.
- Feedback Loops and Nonlinearity: Systems are often characterized by dynamic, nonlinear relationships and feedback loops, which can lead to unexpected and counterintuitive outcomes.
- Emergent Properties: The behavior of a system as a whole can exhibit properties that are not readily apparent from an examination of its individual components.
- Leverage Points: Systems thinkers identify key leverage points – strategic interventions that can have a disproportionate impact on the overall system.
- The systems thinking problem-solving process typically involves the following steps:
- Problem Framing: Defining the problem in the context of the larger system, rather than as an isolated issue.
- System Mapping: Visualizing the key components of the system, their relationships, and the feedback loops.
- Causal Analysis: Exploring the underlying causes and dynamics that shape the system’s behavior.
- Leverage Point Identification: Determining the most effective points of intervention to catalyze positive change.
- Scenario Planning and Simulation: Testing potential solutions through modeling and simulation to anticipate system responses.
By adopting a systems perspective, engineers can develop a deeper understanding of the complex, interrelated nature of the challenges they face. This, in turn, enables them to devise more comprehensive, adaptive, and sustainable solutions that address the root causes of problems, rather than just the symptoms.
The systems thinking approach has been influential in fields such as environmental management, urban planning, healthcare, and organizational development, where the ability to navigate complex, interdependent systems is crucial for driving meaningful and lasting change.
Embracing the Future of Engineering Methods
As the world continues to grapple with an ever-evolving array of complex, interconnected challenges, the engineering methods outlined in this article are poised to play an increasingly pivotal role in shaping the future.
From the continued advancement of computational engineering and the growing emphasis on green engineering, to the integration of systems thinking and the refinement of the engineering method, these problem-solving frameworks will continue to evolve and adapt to the changing landscape of technological, social, and environmental challenges.
Key trends and developments that will shape the future of engineering methods include:
- Technological Convergence: The continued convergence of various technological domains, such as artificial intelligence, robotics, biotechnology, and nanotechnology, will require engineers to adapt their problem-solving approaches to leverage the synergies and interdependencies between these fields.
- Multidisciplinary Collaboration: As the challenges we face become increasingly complex and global in scope, engineering methods will need to embrace a more collaborative, interdisciplinary approach that integrates the expertise and perspectives of professionals from diverse disciplines, including social sciences, policy, and community engagement.
- Emphasis on Sustainability and Resilience: With the growing urgency of addressing climate change, resource depletion, and environmental degradation, engineering methods will place an even greater emphasis on principles of sustainability, circular economy, and resilience to develop solutions that are ecologically responsible and adaptive to changing conditions.
- Embracing Data-Driven and Computational Approaches: The continued advancements in computational power, data analytics, and simulation technologies will further enhance engineering methods, enabling engineers to model complex systems, optimize designs, and make data-driven decisions with unprecedented accuracy and efficiency.
- Prioritizing Ethical Considerations: As the impact of engineered solutions becomes increasingly far-reaching and transformative, engineering methods will need to place a stronger emphasis on ethical deliberation, anticipating potential consequences, and ensuring that solutions align with the principles of social responsibility, inclusivity, and the greater good.
By embracing these emerging trends and adapting their problem-solving approaches accordingly, engineers can position themselves as driving forces in addressing the grand challenges of our time and shaping a more sustainable, equitable, and technologically advanced future for all.
Conclusion
The engineering methods outlined in this article – the scientific method, computational engineering, green engineering, the engineering method, and the systems thinking approach – have been instrumental in driving technological progress, improving human welfare, and addressing the complex challenges facing our world.
As the world continues to grapple with an ever-evolving array of global issues, the versatility and adaptability of these problem-solving frameworks will be crucial in navigating the uncertainties of the future. By embracing the power of these engineering methods, integrating emerging technologies, and fostering interdisciplinary collaboration, engineers can play a pivotal role in shaping a better tomorrow for all.
Through the systematic, analytical, and iterative approaches embodied by these engineering methods, problem-solvers can develop innovative, comprehensive, and impactful solutions that address the interconnected challenges of our time. By leveraging the complementary strengths of these diverse problem-solving frameworks, individuals, organizations, and societies can navigate the complexities of the modern world with greater effectiveness, resilience, and impact.
As we look towards the future, the continued evolution and refinement of engineering methods will be essential in driving progress, fostering sustainability, and enhancing the well-being of both humanity and the planet. By mastering these powerful problem-solving tools, engineers can unlock new frontiers of innovation and make a lasting contribution to the betterment of the world we share.
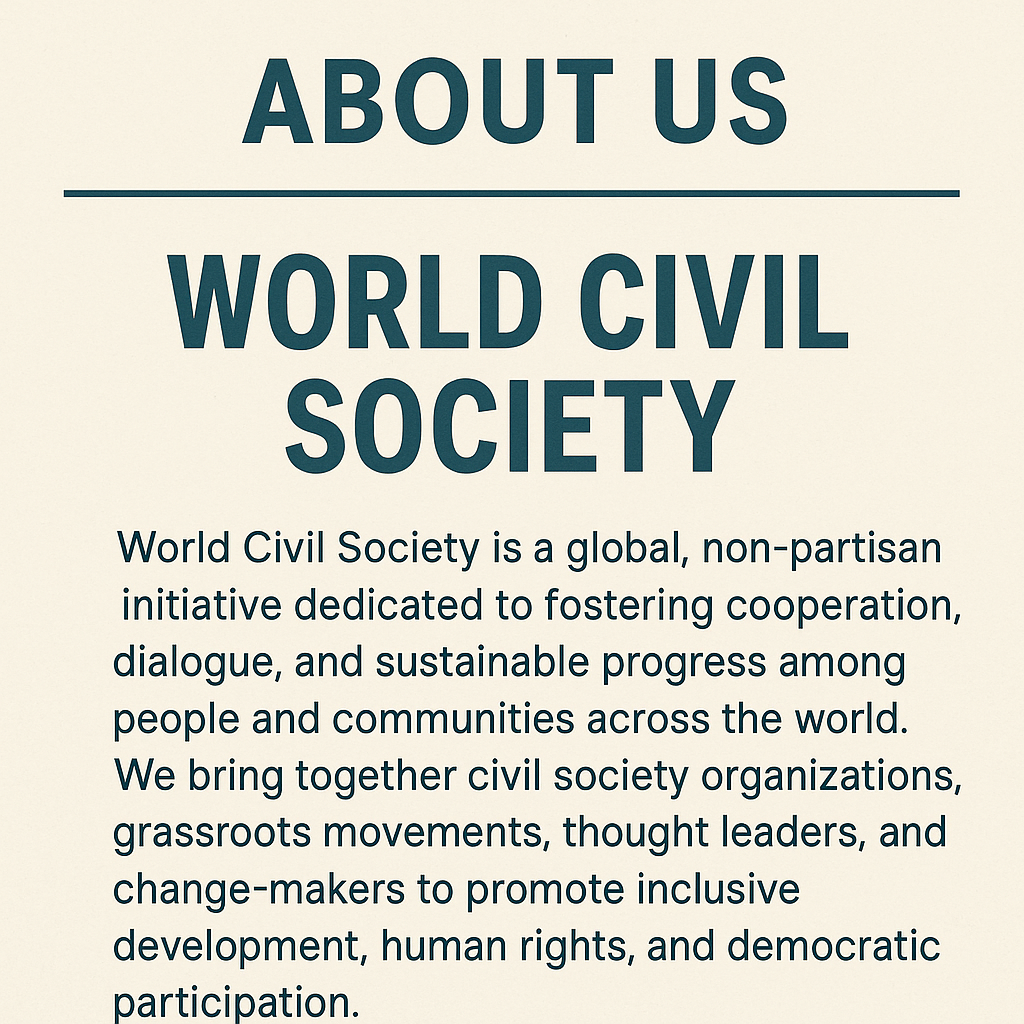
- https://worldcivilsociety.com/different-types-of-engineering/
- https://worldcivilsociety.com/engineering-the-future-tackling-the-four-grand-challenges/
- https://worldcivilsociety.com/the-transformative-power-of-technology-in-engineering/
- https://worldcivilsociety.com/the-engineering-method-a-systematic-approach-to-tackling-complex-challenges/
- https://worldci
- vilsociety.com/mastering-the-art-of-problem-solving-exploring-the-five-essential-methods/
- https://worldcivilsociety.com/mastering-the-art-of-problem-solving-exploring-the-five-essential-methods/
- https://worldcivilsociety.com/navigating-complexity-the-four-approaches-to-effective-problem-solving/
Pingback: The Vital Role of Civil Engineering in Shaping Our Built Environment – worldcivilsociety.com
Pingback: Kenya!!, How much is a civil engineer paid in Kenya?