How to approach an engineering problem?
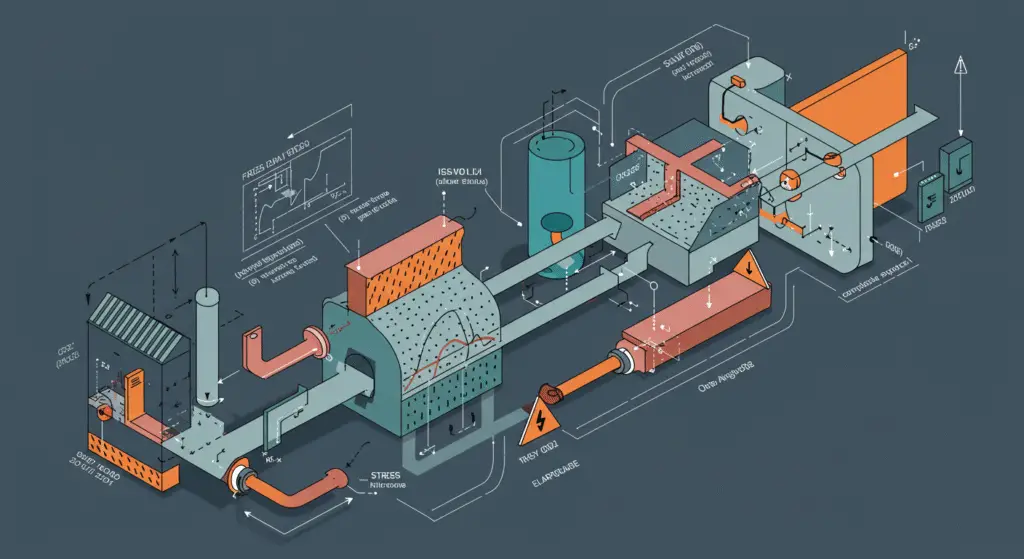
engineering problem
In the dynamic and ever-evolving world of engineering, professionals are routinely faced with complex, multifaceted problems that require a systematic and evidence-based approach to solve. Whether it’s designing a more efficient transportation system, developing a novel medical device, or optimizing an industrial manufacturing process, the ability to effectively identify, analyze, and address engineering challenges is a hallmark of the most successful practitioners in the field.
At the heart of this problem-solving prowess lies a well-defined, step-by-step methodology that guides engineers through the critical stages of understanding the problem, generating potential solutions, and implementing the most viable and impactful design. By mastering this systematic approach to engineering problem-solving, professionals can enhance their critical thinking skills, improve their decision-making abilities, and ultimately deliver innovative and transformative solutions that drive progress and positively impact the world around them.
In this comprehensive article, we will explore the key steps and best practices that define the engineering problem-solving process, equipping you with the knowledge and tools necessary to tackle even the most complex engineering challenges with confidence and success.
Step 1: Define the Problem
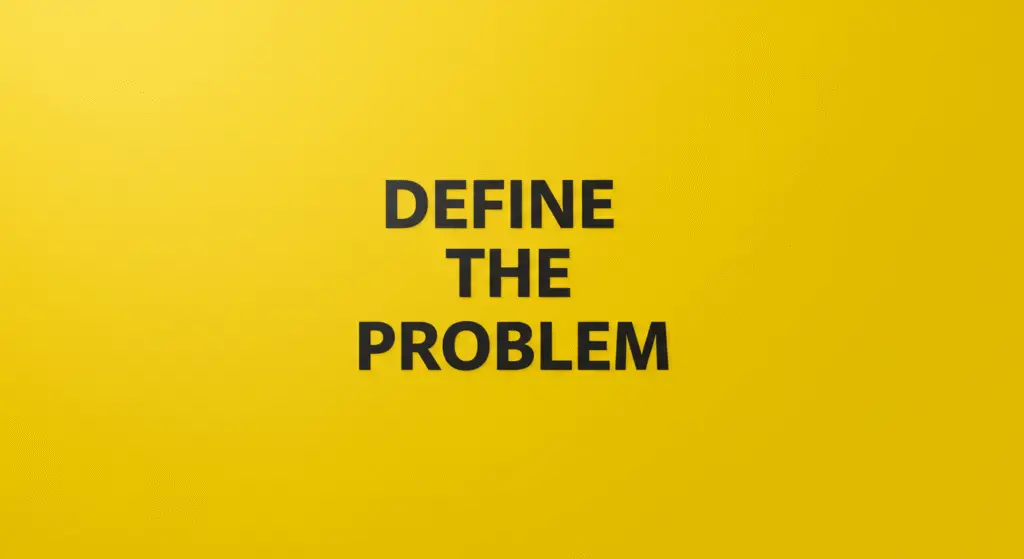
The first and perhaps most crucial step in the engineering problem-solving process is the thorough definition and understanding of the problem at hand. This stage involves carefully examining the challenge, gathering relevant information, and clearly articulating the design objectives, constraints, and requirements.
Key activities in this step include:
- Problem Identification: Clearly defining the problem or opportunity that needs to be addressed, and understanding the context, scope, and stakeholders involved.
- Information Gathering: Conducting comprehensive research to gather relevant data, industry trends, and existing solutions that can inform the problem-solving process.
- Stakeholder Engagement: Engaging with key stakeholders, such as clients, end-users, and subject matter experts, to ensure a deep understanding of their needs, preferences, and pain points.
- Requirement Specification: Defining the specific performance criteria, functional requirements, and design constraints that the final solution must meet.
By investing time and effort in this foundational step, engineers can establish a solid understanding of the problem, align their problem-solving efforts with the needs of stakeholders, and set the stage for the subsequent stages of the engineering problem-solving process.
Step 2: Generate Potential Solutions
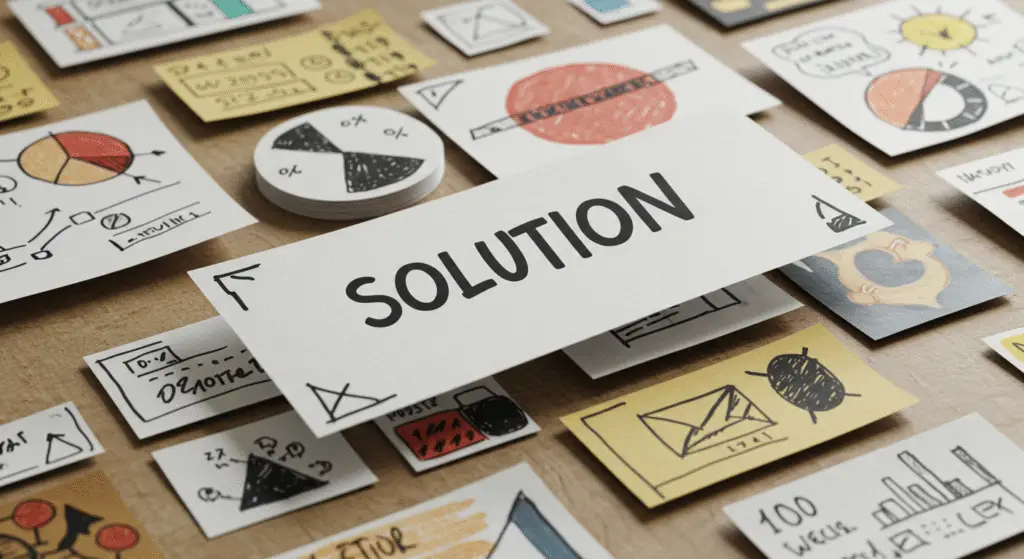
With a clear problem definition in hand, the next step is to explore a diverse range of potential solutions and approaches to problem-solving. This ideation phase encourages creativity, lateral thinking, and the consideration of unconventional ideas.
Key activities in this step include:
- Brainstorming and Idea Generation: Engaging in collaborative brainstorming sessions to generate a wide array of innovative ideas, drawing inspiration from various sources, including past experiences, industry best practices, and out-of-the-box thinking.
- Concept Sketching and Visualization: Translating ideas into visual representations, such as sketches, diagrams, and 3D models, to better communicate and evaluate the proposed solutions.
- Literature Review and Research: Conducting a thorough review of existing research, patents, and industry publications to identify relevant technologies, materials, and design approaches that can inform the development of new concepts.
- Concept Screening and Selection: Systematically evaluating the generated concepts based on the defined requirements, constraints, and selection criteria, in order to identify the most promising solutions for further development.
By embracing a divergent and open-minded approach to ideation and research, engineers can expand the range of possible solutions, challenge existing assumptions, and ultimately identify the most viable and innovative designs to pursue.
Step 3: Analyze and Evaluate Potential Solutions
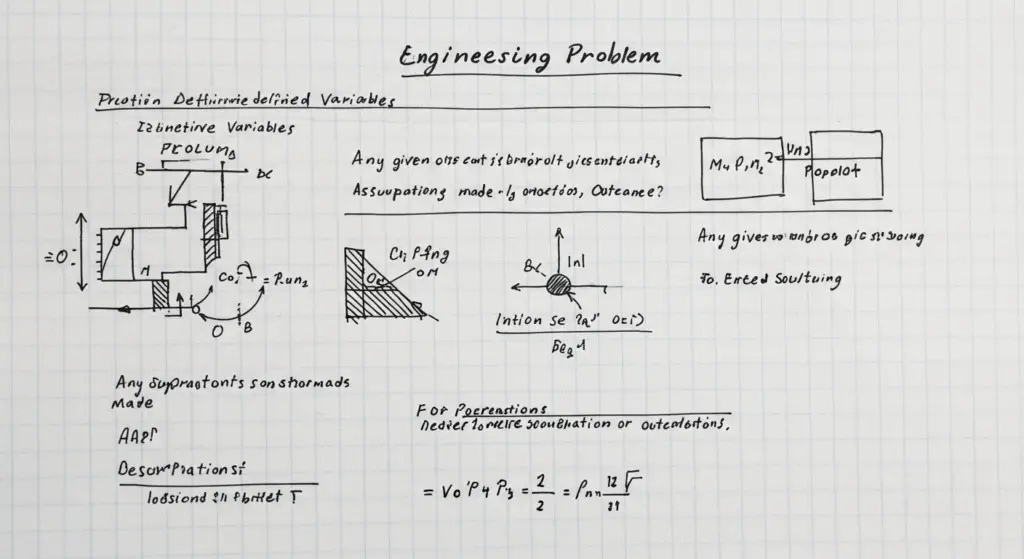
Once a range of potential solutions have been generated, the engineering problem-solving process moves into the detailed analysis and evaluation phase. This step involves the rigorous exploration, modeling, and assessment of the proposed designs, ensuring their technical feasibility, performance, and adherence to the specified requirements.
Key activities in this step include:
- Engineering Calculations and Simulations: Performing detailed engineering calculations, computational modeling, and simulations to analyze the design’s structural integrity, material properties, thermodynamic performance, and other relevant parameters.
- Prototyping and Testing: Constructing physical or digital prototypes to validate the design’s functionality, identify potential issues, and gather empirical data for further optimization.
- Failure Mode and Effects Analysis (FMEA): Systematically examining potential failure modes, their causes, and their effects, in order to identify and mitigate risks associated with the design.
- Comparative Analysis and Evaluation: Assessing the relative merits of the proposed solutions based on the defined criteria, such as performance, cost, feasibility, and sustainability, to identify the most promising design.
By meticulously analyzing and evaluating the potential solutions, engineers can ensure the final design meets or exceeds the specified requirements, while also identifying opportunities for further improvement and optimization.
Step 4: Select the Optimal Solution
With a range of potential solutions analyzed and evaluated, the engineering problem-solving process moves into the critical phase of selecting the most viable and promising design. This step involves a comprehensive evaluation of the alternatives, considering both quantitative and qualitative factors, to identify the solution that best meets the defined requirements and objectives.
Key activities in this step include:
- Evaluation Criteria Development: Establishing a set of criteria, such as performance, cost, feasibility, and sustainability, that will be used to assess the relative merits of the different solutions.
- Solution Comparison and Ranking: Systematically comparing the proposed solutions against the established criteria, using weighted scoring matrices or other decision-making tools to identify the design that best addresses the problem.
- Stakeholder Feedback and Input: Engaging with key stakeholders, such as clients, end-users, and subject matter experts, to gather their feedback and perspectives on the proposed solutions, ensuring the selected design aligns with their needs and expectations.
- Final Solution Selection: Finalizing the selection of the most promising design, based on the evaluation results and stakeholder input, and preparing to move forward with the detailed development and implementation of the chosen solution.
By rigorously evaluating the available options and incorporating stakeholder feedback, engineers can ensure the selected design offers the optimal balance of performance, feasibility, and alignment with the problem’s requirements.
Step 5: Implement and Validate the Solution
The final step in the engineering problem-solving process involves the deployment of the designed solution, its validation in real-world conditions, and the collection of feedback from stakeholders to inform future improvements and iterations.
Key activities in this step include:
- Implementation Planning: Developing a detailed implementation plan that outlines the necessary resources, timelines, and workflows for the successful deployment of the solution.
- Deployment and Validation: Overseeing the successful deployment, installation, and commissioning of the final product or system, ensuring it is integrated seamlessly into the target environment and meets the specified requirements.
- Performance Monitoring and Evaluation: Continuously monitoring the solution’s performance, collecting data on its efficiency, reliability, and user satisfaction, and identifying any issues or areas for improvement.
- Stakeholder Feedback and Review: Actively engaging with end-users, clients, and other stakeholders to gather feedback on the solution’s performance, usability, and alignment with their needs and expectations.
- Design Iteration and Continuous Improvement: Incorporating the insights gained from the deployment and feedback phases to refine the design, address any identified issues, and prepare for future generations or iterations of the solution.
By actively evaluating the performance of the deployed solution and incorporating stakeholder feedback, engineers can ensure the design continues to meet the evolving needs of the end-users, while also laying the groundwork for future improvements and innovations.
The aIterative Nature of Engineering Problem-Solving
It is important to note that the engineering problem-solving process is not a linear, sequential series of steps, but rather an iterative and interconnected framework. As engineers navigate this process, they may find themselves revisiting previous stages, refining their understanding of the problem, or exploring alternative solutions based on new insights or changing requirements.
This iterative nature of the engineering problem-solving process is a critical aspect of its effectiveness, as it allows engineers to continuously learn, adapt, and improve their designs in response to the complex and dynamic challenges they face. By embracing a mindset of continuous improvement and a willingness to explore alternative paths, engineers can enhance the quality, reliability, and impact of their solutions.
Moreover, the interconnectedness of the various steps in the engineering problem-solving process highlights the importance of holistic thinking and the ability to navigate the complex web of technical, operational, and stakeholder considerations that shape the success of a design project.
Mastering the Engineering Problem-Solving Process
Mastering the engineering problem-solving process is a crucial skill for professionals in the field, as it equips them with a systematic, evidence-based approach to tackling even the most complex challenges.
By developing a deep understanding of the five steps outlined in this article, engineers can cultivate the following key competencies:
- Critical Thinking and Problem-Solving: The engineering problem-solving process fosters the ability to analyze complex problems, identify underlying issues, and devise innovative, evidence-based solutions.
- Collaboration and Communication: The process often requires close collaboration with cross-functional teams, stakeholders, and subject matter experts, thereby honing an engineer’s communication and interpersonal skills.
- Adaptability and Continuous Learning: The iterative nature of the engineering problem-solving process encourages engineers to remain flexible, open-minded, and committed to continuous improvement and professional development.
- Project Management and Execution: The process provides a structured framework for planning, organizing, and executing engineering projects, ensuring they are delivered on time, within budget, and to the required specifications.
- Technical Expertise and Innovation: By applying the engineering problem-solving process, engineers can leverage their technical knowledge and expertise to push the boundaries of innovation and contribute to the advancement of their respective fields.
As the world continues to grapple with complex, multifaceted challenges, the ability of engineers to navigate the problem-solving process with skill, agility, and a commitment to the greater good will become increasingly crucial in shaping a better, more sustainable future for all.
Conclusion
The engineering problem-solving process, with its five critical steps – defining the problem, generating potential solutions, analyzing and evaluating solutions, selecting the optimal solution, and implementing and validating the solution – is a powerful framework that empowers professionals to tackle even the most daunting engineering challenges.
By mastering this systematic approach to problem-solving, engineers can cultivate a diverse array of essential skills, from critical thinking and collaboration to technical expertise and innovation. Moreover, the iterative and interconnected nature of the engineering problem-solving process encourages a mindset of continuous learning and improvement, equipping engineers with the adaptability and resourcefulness needed to navigate the ever-evolving landscape of technological advancement and global challenges.
As the world continues to place greater demands on the engineering profession, the ability of professionals to leverage the problem-solving process to develop innovative, evidence-based solutions will become increasingly crucial in shaping a more sustainable, resilient, and prosperous future for all. By embracing this powerful framework, engineers can unlock new frontiers of progress, drive positive change, and leave an indelible mark on the world around them.
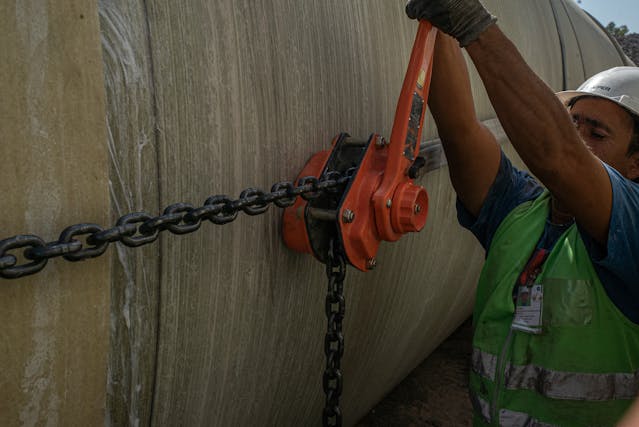
- https://worldcivilsociety.com/navigating-the-engineering-design-process-a-comprehensive-guide-to-innovative-problem-solving/
- https://worldcivilsociety.com/the-vital-realm-of-civil-engineering-shaping-the-built-environment-and-beyond/
- https://worldcivilsociety.com/civil-engineering-landscape-exploring-salaries-and-career-prospects-in-kenya
- https://worldcivilsociety.com/the-vital-role-of-civil-engineering-in-shaping-our-built-environment/
- https://worldcivilsociety.com/unlocking-innovation-the-transformative-power-of-engineering-methods/